Much attention is focused today on pathogenic microorganisms that have developed resistance to antibiotic treatment, or entire types or classes of antibiotics. The loss of effective antibiotic treatment undermines the ability of healthcare professionals to fight infectious diseases and manage their complications among immunocompromised patients.
The Centers for Disease Control and Prevention (CDC) estimates that more than two million people in the United States are sickened every year with antibiotic-resistant infections, with at least 75,000 dying (2013) as a result. Healthcare associated infections (HAIs) kill more people in this country than AIDS, breast cancer and auto accidents combined.
Healthcare associated infections are also known as nosocomial infections or hospital-acquired infections. They are transmitted by a variety of vectors, including person-to-person, through injection/insertion of medical devices, airborne contact of open wounds, and by respiration of airborne particles. Some emerging diseases, such as Middle East Respiratory Syndrome (MERS) are not yet understood well enough to positively identify the transmission vector.
The most dangerous HAI pathogens are those that have the potential to spread by the airborne route (Kowalski 2006). Many of these pathogens, such as Methicillin-resistant Staphylococcus aureus (MRSA), are now called “superbugs” because they are virtually invincible to standard drug treatments. Favorable indoor environments tend to self-perpetuate these agents, adding to the concern by infection control specialists everywhere.
According to the CDC and World Health Organization, antibiotic-resistant HAIs are on the rise. Support for airborne disease transmission is also on the rise (Fletcher et al. 2003). Evidence exists for airborne nosocomial transmissions of Acinetobacter, Pseudomonas, and MRSA (Allen and Green 1987), (Ryan et al. 2011) and (Farrington et al. 1990), and airborne transmission can spread rapidly and pervasively through a non-immune population (Weinstein 2004).
If mechanical and functional operations have remained unchanged, other sources of drug-resistant contamination must exist, presumably associated also with allusive paths of transmission. Therefore, source and pathway management should involve airborne transmission and especially enhanced methods of its control even though the primary route is considered to be direct contact. This article discusses several control methods.
Infection controls
Addressing infection control in hospitals requires integrating HVAC and air-pressure-control with dedicated infection-control systems, and minimizing unplanned airflows through building envelopes and interior spaces. It also benefits from the application of ultraviolet UV-C equipment from what is typically referred to in the healthcare industry (and the CDC) as ultraviolet germicidal irradiation (UVGI).
There are four methods used to reduce the concentrations of airborne infectious agents: dilution, filtration, pressurization, and disinfection. Following is a brief discussion of each method, with a focus on disinfection.
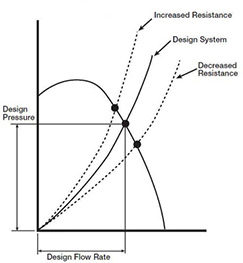
Dilution
Dilution ventilation helps to control infectious particles by introducing outdoor air, usually 2 to 5 air changes/hour (ACH), to dilute space air and then exhausting that amount as contaminated air. If 100 percent of all supply air were outdoor air, nearly that amount of airborne infectious particles might be exhausted. However, conditioning that amount of outdoor air would be cost prohibitive and therefore considered out of the question.
Filtration
The combination of filtration equipment and airflow rates are often misunderstood or underappreciated for the effect they have on the concentration of infectious agents in any conditioned space. Filtration should be considered healthcare’s first line of defense against infectious agents as it removes a large percentage of them with every complete air change through an air handler. If the filter efficiency and/or air change rate is increased, a larger number of infectious agents would be removed per pass.
Therefore, it’s important to view the return air and filtration system as a removal method of space generated contaminants - and not the air distribution side as a pathogen source, with the possible exception of some smaller viral particles. If there is concern here, the application of UV-C in the air handler equipment is warranted.
Current design guidelines suggest air change rates up to 25 (up to five of them as outdoor air) for new facilities, depending on the space served. Because most of the airborne pathogens originate from patients and room occupants, increasing the rates of supply air above design guidelines will bring diminishing returns. Thus, the incremental benefit in preventing cross-transmission is much more difficult to demonstrate beyond 25 ACH. This can be seen in this simple equation:
Concentration (particles/cu.ft)= generation rate (no. of people/activity)
cfm x filter eff. (removal rate)
If either the cfm or filter efficiency is increased, the particle concentrations will decrease mathematically. However, the algorithm favors reducing in-room source or generation rates of infectious agents, often referred to as source control or source reduction.
Pressurization
Pressurization protects against cross contamination from the infiltration of air from one space type to that of another. This is of great importance in healthcare settings, but it is very difficult to control. Frequently opened or propped open doors are all too common making corridors, etc., a conduit of contaminated air to other spaces. Although ORs and other areas are designed to be under positive pressure with respect to external spaces, this may not be the case when an air handler’s airflow has been compromised!
Note that air handler design resistance is the sum of all pressure losses through the system, including elbows, dampers, filters and coils, etc. The shape of a resistance curve will change when pressure losses change (Greenheck 1999). For instance, as filter resistance increases, system air volume is reduced. But, like the filter, it’s also common for the coil pressure drop to increase, even double, which will result in a higher system pressure drop as well. The curves show that as the system’s total resistance increases, air volume and system pressure capability are reduced (at a constant fan RPM). This reduction in ‘design-pressure’ occurs often today from reduced coil cleaning procedures, but its effect is not at all obvious, and it’s not just a reduction in air volume, although that’s important, it’s also a reduction in relative room pressure capability! When one space is said to be negative in relation to other spaces, it assumes that the adjoining spaces are all ‘positive’. A higher coil pressure drop will potentially negate that and permit the infiltration of contaminated air.
Measuring the pressure drop across a coil and comparing it to as-built design data, or better, the manufacturer’s coil performance data, is one way to determine a potential loss in airflow. If the coil pressure drop is higher, the actual airflow should be measured to confirm that it matches as-built criteria. If the coil is fouled (common) and air pressure compromised, the 2011 ASHRAE Handbook – HVAC Applications recommends the installation of UV-C lamps to clean the coil and keep it clean continuously. A clean coil will assure proper airflow and pressure relationships with the added benefit of restoring as-built cooling capacity (i.e., the heat-transfer characteristics). According to ASHRAE, UV-C will also eliminate the growth of coil plenum mold and bacteria removing the possibility of microbial products carryover and transfer to conditioned spaces.
Disinfection
In addition to opening and propping open of doors as a contaminant transfer mode, the entering and exiting of people also provides a contaminant source. It’s known that the concentration of airborne bacteria is proportional to the number of personnel in the room (Mangram et al. 1999, Duvlis and Drescher 1980, Moggio et al. 1979, Kundsin 1976). The amount of surface contamination is also related to airborne contamination from occupation and activity since these microbes settle continuously. The World Health Organization (WHO 1998) recommends a limit of 100 cfu (colony forming units)/m3 for bacteria and 50 cfu/m3 for fungi for sensitive areas. There are no published cfu standards in the U.S.
While the use of UV-C equipment, or disinfection, to control infectious agents in healthcare settings is one of its oldest uses, today the technology is under-utilized. In the past seventy years it has used to disinfect upper air, ventilation air, and to sterilize medical equipment and water, with measured and successful results. However, its use waned as dependence on antibiotics began in the late 1950s and beyond. UV-C destroys all microorganisms… and its use is extremely simple and inexpensive, but like all controls, it alone is not a complete answer.
UV-C for infection control
There are three primary means of applying UV-C systems against infectious agents: upper-air (upper-room), coil irradiation, and airstream disinfection. Upper-air systems are installed in room spaces, such as above patient beds and in waiting rooms, corridors and break areas, etc. Coil irradiation and airstream disinfection systems are installed within air handling units or duct runs. Upper room and HVAC applications are described below.
Upper Air/Room
The primary objective of upper-air UV-C placement is to interrupt the transmission of airborne infectious diseases in patient rooms, waiting rooms and other known microbial pathways such as lobbies, stairwells, laundry chutes, and emergency entrances and corridors, all of which can be effectively and affordably treated with UV-C (ASHRAE 2011). Airborne droplets containing infectious agents can remain in room air for 6 minutes and longer. Upper Air UV-C fixtures can destroy those microbes in a matter of seconds. Operating 24 hours a day, upper-air systems are also especially effective at notably reducing the potential viability of surface microbes that settle out of room air.
Humans are the source of airborne agents which infect people (Nardell and Macher: ACGIH 1999). Again, upper-air systems intercept microbes where they are generated, thereby controlling them at the source (First et al. 1999). They have been shown to be effective against viruses and bacteria, including chickenpox, measles, mumps, varicella, TB, and cold viruses. Studies of Mycobacterium tuberculosis have shown that they can be equivalent to 10–25 air changes per hour (CDC 2005). In a study by Escombe et al. (2009), guinea pigs were exposed to exhaust air from a TB ward, of which 35 percent of the controls developed TB infections while only 9.5 percent developed infections where upper air UV-C was used, yielding a decrease of 74 percent in the infection rate.
Measles and influenza viruses and the tuberculosis bacteria are diseases known to be transmitted by means of shared air between infected and susceptible persons. Studies indicate that there are two transmission patterns: (I) within-room exposure such as in a congregate space; (II) transmissions beyond a room through corridors, and through entrainment within ventilation ductwork where air is then recirculated throughout the building. Since the 1930s (Wells 1955; Riley and O’Grady 1961) and continuing to the present day (Miller et al. 2002; Xu et al. 2003; First et al. 2007), numerous experimental studies have demonstrated the efficacy of upper-air UV-C. In addition, effectiveness has been shown for reducing measles transmission in a school, and influenza transmission within a hospital (McLean 1961). What’s more, newer fixtures available today provide more output and coverage at less cost and power. They also utilize inexpensive and commonly available lamps!
HVAC systems
HVAC systems provide an excellent growth area for mold and some bacteria in and around cooling coils; drain pans (Levetin et al. 2001), plenum walls and filters. Growth of these microbial deposits also leads to coil fouling which will increase coil pressure drop and reduce airflow and heat exchange efficiency (Montgomery and Baker 2006). As performance degrades, so does the quality, amount and pressurization capability of air supplied to conditioned spaces (Kowalski 2006/2009).
Because hospital codes call for high-efficiency filters to be located downstream of the cooling coil, they can also become damp and often wet from saturated air in that location. As such, air filters are considered a growth medium for mold and bacteria and an infectious-disease agent reservoir. ASHRAE recommends UV-C lighting to be installed downstream of the cooling coil; so if a 360 degree UV-C system is installed there, it will disinfect both the coil and the filter to destroy all microbes in and upon both devices. It should also be noted that when using a 360-degree lamp in a “common” coil-irradiation system, it will also kill infectious diseases in the airstream. For example, up to a 35 percent kill ratio of many infectious agents is achieved, thus providing a measurable increase in the combined removal rate of the two devices (Kowalski 2009).
UV-C design guidance
Importantly, science has not found a microorganism that is resistant to the destructive effects of the 254-nm germicidal wavelength, including superbugs and all other microbes associated with HAIs. The question then – how are UV-C systems sized and applied?
Historically, engineers and facility practitioners wanting to apply UV-C lacked specific guidance for systems design, sizing and specifications. ASHRAE undertook the process by forming a technical committee (TC 2.9 Ultraviolet Air and Surface Treatment) to author chapters in their 2008, 2011, and 2012 ASHRAE Handbooks, which have been referenced herein. HVAC trade publications have also published several technical articles to help provide additional design guidance; these articles are cited in the sidebar, Technical Articles for Engineers. In total, these articles provide all practitioners with the guidance needed to successfully design, install, operate, and maintain successful UV-C applications in HVAC systems.
UV-C at large
UV-C's rising popularity beyond ASHRAE has also generated research by lesser-known organizations, such as the Air Purification Consortium (APC); the Air Cleaning Industry Expert Advisory Panel (ACIEAP); and The National Center for Energy Management and Building Technologies (NCEMBT). UV-C energy has been crucial to achieving each of their goals, whether to save energy, reduce biological contamination and maintenance or to reduce absenteeism. Their members are involved in high-stakes projects such as Homeland Security where application of UV-C is a crucial defense against bioterrorism.
Safety & handling
Opening air handler doors to fan sections must be minimized because it allows unfiltered air to enter and be dispersed to potentially sensitive areas, and/or it will disrupt pressure relationships in the spaces served by them. Shutting these systems down can also disrupt pressure relationships beyond the spaces served. Both of these functions, when necessary, should be coordinated with floor nurses so that all room doors may be closed before hand. Exposing filter surfaces to UV-C is an effective way to destroy microbes on media surfaces. However, synthetic media filters are not compatible with UV-C while filters with glass media are. Caution should also be exercised when using unsupported “bag” style filters as they inherently collapse when being replaced to expel potentially microbe- laden contamination. The CDC also recommends that all used filters be bagged upon removal to prevent dispersion of microbial contamination during transport.
Where installed, facility staff should be trained how to inspect UV-C systems to ensure they are working properly. Controls should be installed to turn UV-C systems off when air-handler doors are opened. Eye and skin protection are needed to prevent exposure to UV-C light when working in any area where the lamps are on.
UV-C lamps are very similar in construction to fluorescent lamps, and therefore contain trace amounts of mercury. The use of encapsulated lamps is recommended to prevent air-handler contamination should lamp breakage occur. Like fluorescent lamps, UV-C lamps should be replaced and recycled annually in a scheduled fashion.
Conclusion
UV-C installations are a simple, effective, and relatively inexpensive means of reducing concentrations of airborne and surface pathogens that cause healthcare associated infections. Within patient rooms, waiting rooms, and other congregational areas, upper-air UV-C units will kill airborne microorganisms that inherently circulate into the path of the UV-C light. UV-C lamps can be installed within HVAC systems downstream of cooling coils to keep coils clean and to provide supplemental kill ratios in airstreams and on filter surfaces. Recent guidance from ASHRAE and published technical articles in HVAC trades provide healthcare engineers and facility staff with the resources needed to size, select, install, operate, and maintain UV-C systems.
Design considerations
• Concentration of airborne infectious agents is directly related to people activity
• Humans are the source of drug resistant microorganisms that effect humans
• Airborne transmission of infectious agents may be more prevalent than proven
• UV-C inactivates and destroys microorganisms rendering them harmless
• 70+ year old Upper Air UV-C technology is heavily researched and proven effective
• Newer Upper Air UV-C units are more affordable and much more effective
• At 6 ACH an aerosol of infectious agents can stay airborne for 10 minutes
• Upper Air UV can inactivate airborne infectious agents in a matter of seconds
• Source management will always prove to be the most effective means of control
• Increased coil pressure drop will lower system airflow and space pressurization
• Bathing coils with UV-C cleans them, improves airflow and heat transfer efficiency
Additional Tips
• Install UV-C on cooling coils and drain pans to kill mold and restore airflow
• Manage room pressure relationships, especially during visiting hours
• Review and manage all air handler service, especially filters and change-outs
• Manage air handler shutdowns, access door openings and coil pressure drop
• Install newer style upper air UV-C fixtures in all spaces known for HAI’s
• Also install them in corridors and waiting rooms connected to these areas
• Once airflow is restored, upgrade air filters and efficiencies where possible
Forrest Fencl is the president of UV Resources, Santa Clarita, Calif. He can be reached at forrest.fencl@uvresources.com.